Story at a glance:
- Passive house is a set of voluntary building standards that aims to drastically improve the energy efficiency of a built structure through the use of passive building strategies.
- Both the Passivhaus Institute and Passive House Institute US (PHIUS) promote and certify passive building projects in the United States.
- Principles of passive house design include using superinsulation, eliminating thermal bridges, installing high-efficiency windows, and using effective air-sealing techniques and heat recovery ventilation systems.
People have been implementing passive design elements since the construction of the earliest built shelters, but the passive house concept in sustainable building design is a much more recent innovation. Since its origin in Europe during the late 1980s, the ideology and techniques defining the passive house concept have revolutionized the way architects, engineers, and developers approach, plan, and design sustainable, high-efficiency buildings.
Here are the basics and history of passive house standards, the principles that define passive house design, and a few real-world examples.
What is Passive House?
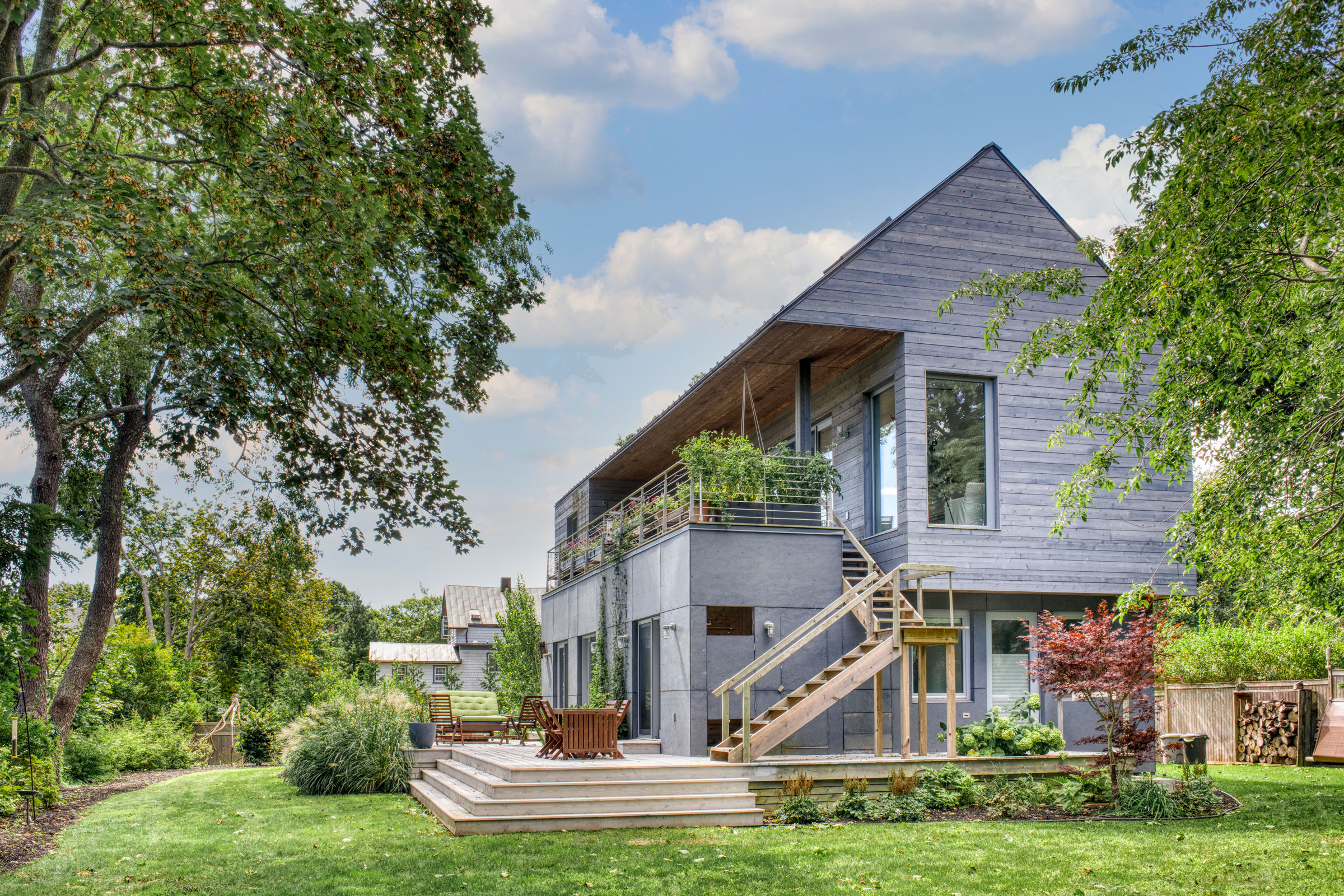
Wayne Turett, founder and principal of The Turett Collaborative, designed and constructed his own passive house in Greenport, New York. Photo by Liz Glasgow
In architecture the term “passive house” refers to a voluntary set of building standards and principles that seek to maximize the use of passive building strategies to both reduce a structure’s energy use and provide a comfortable indoor environment year-round. Passive house designs typically use 80% less energy for heating and cooling, are much healthier, and are better at regulating interior temperatures than conventional buildings.
Buildings designed to the passive house standard are defined by their use of the following building-science strategies, as identified by the Passive House Institute US (PHIUS), the leading certifier of passive house projects in the United States.
- Use of continuous insulation that minimizes or eliminates thermal bridging.
- Construction of an extremely airtight, durable, and resilient envelope.
- Installation of high-performance double- or triple-paned windows.
- Implementation of a balanced heat-and-moisture recovery ventilation system.
- Minimization of space conditioning system because of lower space conditioning loads.
Exact criteria and performance measurements for achieving Passive House certification from PHIUS depends partially on the building’s classification, identified as either Single-Family, Multi-Family, Office, School, or Other Non-Residential; the full guidebook and all requirements may be accessed via their webpage.
A Brief History of Passive House Standards

Photo courtesy of PHIUS
First coined in the early 1970s, the term “passive house” was originally used to describe those homes designed to exploit the use of passive solar strategies and minimize heating and cooling energy requirements.
It was not until 1988 that “passive house”—or passivhaus, to some—began being used to refer to a set of building standards, originating out of a conversation between Wolfgang Feist of the Institut für Wohnen und Umwelt and Bo Adamson of Sweden’s Lund University. By 1991 the first modern “passivhaus” had been built in Darmstadt, Germany—its construction characterized by the use of superior insulation, improved airtightness, more efficient windows, and a carefully controlled ventilation system.
In 1996 the Passivhaus Institut (PHI) was founded in Germany to validate, promote, and control these passive house standards. Building to these standards would start to become popular throughout Europe during the 2000s, with more than 15,000 buildings either built or remodeled to PHI standards by 2010. It’s estimated that there are now more than 40,000 passive house projects across Europe, with more being constructed every year.
There are comparably few buildings built to passive house standards in the United States, but it is has become considerably more commonplace, with more than 39,000 projects certified within the last two decades. This is largely thanks to the efforts of PHIUS, although both PHI and PHIUS promote and certify passive house projects in the United States. PHIUS operates independently from PHI, however, and created its own standard, PHIUS+, in 2015.
Benefits of Building to Passive House Standards
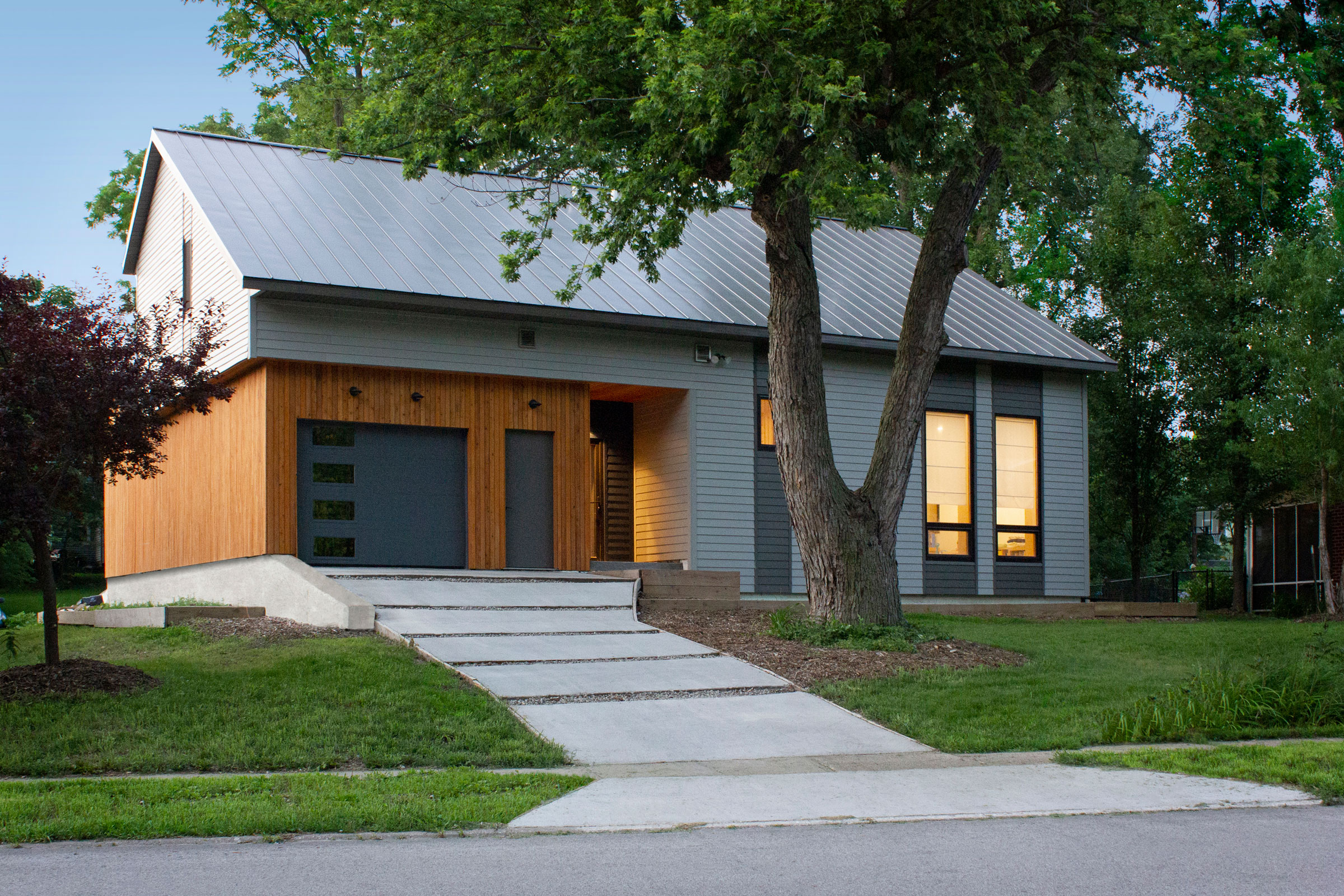
The Iowa City Nest residence is an all-electric, low-carbon passive house. Photo by Carl Sterner
It’s no secret that building to passive house standards greatly reduces a structure’s overall energy consumption, but there are a range of additional benefits that Passive House-certified buildings also enjoy.
Energy Efficiency & Reduced Operating Costs
Structures built to passive house standards are, by their very nature, designed to be as energy-efficient as possible. In fact, most Passive House-certified buildings use only 10 to 25% of the energy that buildings built to conventional standards use for their heating and cooling needs. This improved energy efficiency is not only good for the environment but translates to a lifetime of drastically reduced operating costs for the building’s owner, allowing them to potentially save thousands each year on utility bills.
Improved Indoor Air Quality
One of the hallmarks of passive house design is the implementation of a highly effective ventilation system that, when combined with superior air-sealing, greatly improves the overall air quality of the indoor environment. These qualities help ensure that buildings built to passive house standards provide adequate air circulation, maintain stable humidity levels, and minimize the entry of dirt, dust, pollen, mold spores, or other small particulate matter that might cause or trigger respiratory illnesses or afflictions.
Reduced Environmental Footprint
Because passive house standards seek to greatly reduce a structure’s electricity usage and overall dependency on energy produced through the burning of fossil fuels, they also help prevent a significant amount of GHG emissions from being released into the atmosphere. Combined with their durability and superior longevity, passive structures have a much smaller environmental footprint than buildings constructed to conventional standards.
Comfortable Indoor Environment
Designing to passive house standards has the additional benefit of cultivating a comfortable indoor environment that naturally and effectively regulates temperature, airflow, and humidity levels throughout the year, with minimal need for mechanical systems. The superior insulation and air-sealing of Passive House-certified homes not only ensures thermal comfort, but also provides improved noise control as well, creating a quieter indoor environment with less distractions.
Resilient & Durable
Due to their careful planning, rigorous testing, and use of quality materials, buildings that are designed to passive house standards are more durable and resilient towards extreme weather events compared to conventionally-built buildings. This ultimately translates to long-term cost savings when it comes to maintenance and repairs while also reducing the amount of construction waste sent to landfills throughout the building’s lifespan.
Challenges of Passive House Standards
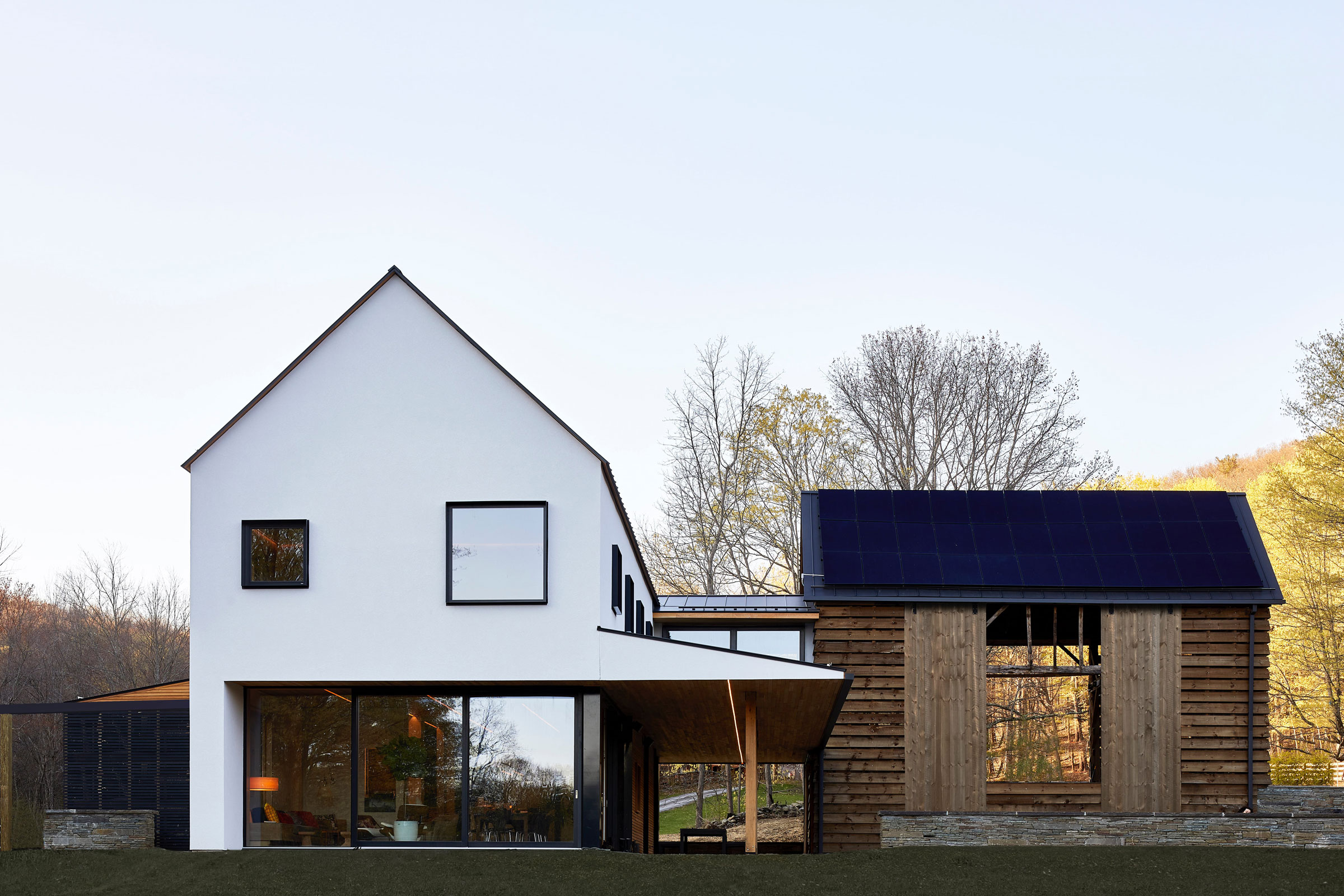
Gallatin Passive House. Photo courtesy of the 2021 PHIUS Passive Projects Design Competition
Building to passive house standards comes with many benefits, but there are also a few challenges that can make passive house projects difficult to achieve.
Higher Upfront Costs

Photo courtesy of Phoenix Haus
Building to the passive house standard has become considerably less expensive over the last decade, but it still costs more than building to conventional building standards. Most buildings built to comply with passive house standards come with a 3 to 5% cost premium.
These higher upfront costs are ultimately justified by the amount saved in operating costs over the course of the building’s lifespan. “When a cost analysis is performed and this premium is amortized over the life of the structure, it turns out to be a marginal increase in the investment that start paying you back the minute you start living in the structure,” Kate McDonald, project manager at Phoenix Haus—a prefab home manufacturer whose designs incorporate Passive House principles—told gb&d in a previous article.
Regulatory Barriers
Building codes and regulations have come a long way with regard to HVAC energy efficiency and minimum insulation requirements, but there’s still a noticeable lack of regulations in support of or incentivizing the adoption of passive house design principles.
This is largely because most code development adoption processes operate on a three-year schedule and require all proposed changes to pass a cost-effectiveness test in isolation before they are integrated into the new code—a practice that can hinder the adoption of highly interconnected, holistic passive house principles that work in conjunction with one another.
Some decision-makers in the code adoption process may also not support the updating of energy efficiency code standards.
Lack of Experienced Professionals
Another challenge surrounding the adoption of passive house strategies is the general lack of education and experience in the field itself, particularly among AEC professionals and the general population. Despite the growing popularity of passive house certification, there’s a comparatively low number of designers, architects, engineers, and construction companies with experience with passive house techniques and technologies.
There are also a few misconceptions about passive house projects, especially when it comes to cost. While it’s true that designing to passive house standards can have more upfront costs than conventional standards, many developers and planners overestimate those costs or intentionally misrepresent those to keep the status quo from changing in favor of more sustainable building construction.
Principles of Passive House Design

Photo courtesy of PHIUS
To achieve optimal comfort and minimize energy usage, passive house design prioritizes the following principles: thermal control, air control, and solar radiation control.
Thermal Control
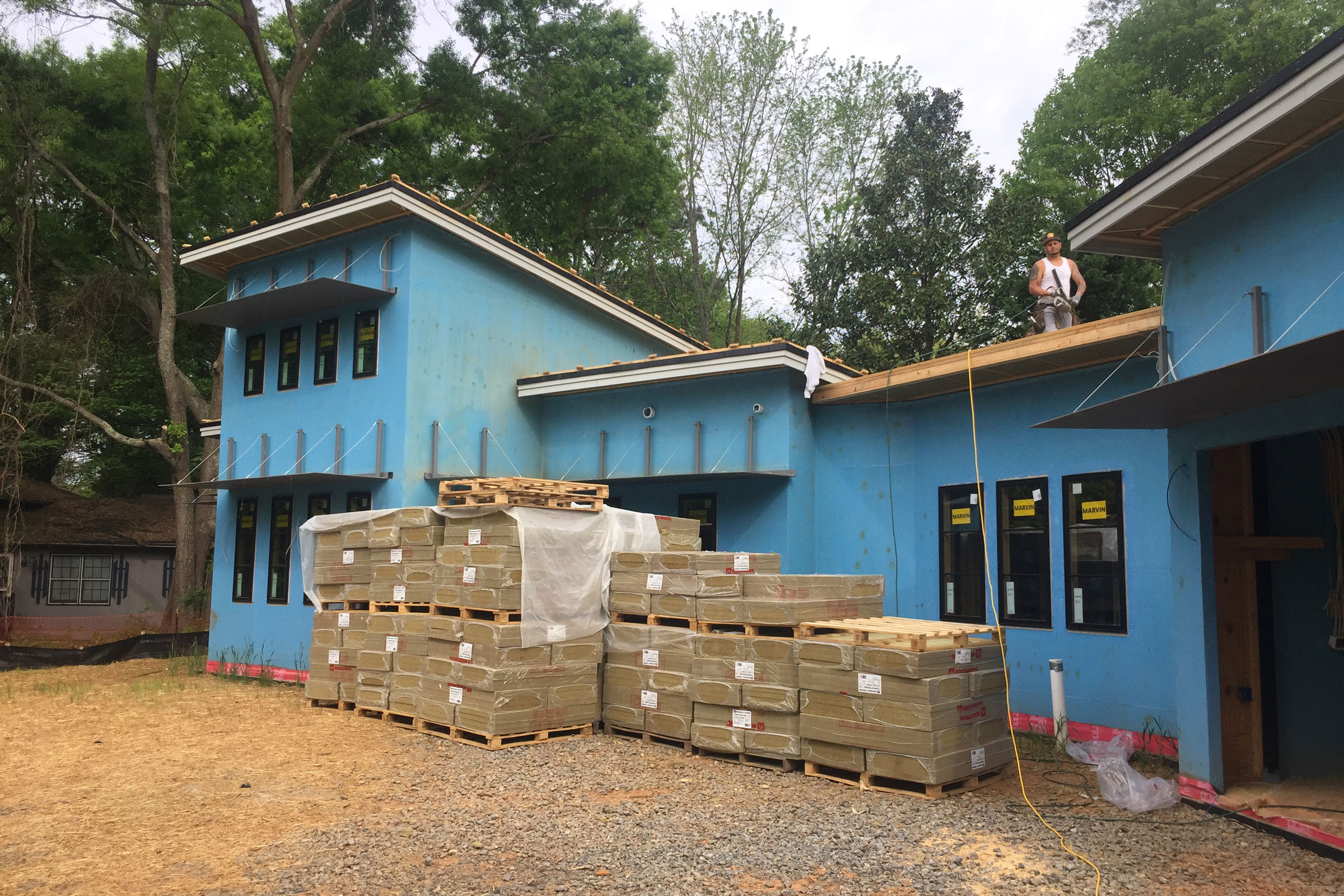
Thermal insulation plays a key role in making a home more energy-efficient by helping to reduce the energy required for heating/cooling the house. Photo courtesy of LG Squared
As perhaps the most important factor in passive house design, thermal control refers to a building’s ability to effectively prevent heat from entering or exiting as a result of heat transference (or conduction) through the materials used in its construction. Thermal control is achieved through the use of two key methods: superinsulation and the elimination of thermal bridges.
Superinsulation
All buildings require some amount of thermal insulation in order to comply with local codes and regulations, but passive house standards go far beyond the minimum required amount by implementing what’s known as superinsulation. First coined in 1976 by Wayne Schick at the University of Illinois Urbana-Champaign, “superinsulation” is a term generally used to describe insulation with a very high R-value—typically R-40 for walls and R-60 for roofs—and is a staple of passive house construction.
When combined with an airtight envelope and heat recovery ventilation systems, superinsulation allows a structure to rely primarily on intrinsic heat sources (e.g. body heat of occupants and waste heat from appliances), greatly reducing the need for supplementary mechanical heating. Installing superinsulation—typically in the form of continuous rigid exterior insulation—is also one of the easiest methods for retrofit projects to achieve Passive House certification.
Elimination of Thermal Bridges
It is not uncommon for buildings to have several instances of thermal bridging, or areas in which there is a direct connection between the interior and exterior through building components that possess a higher thermal conductivity than those materials surrounding them. Thermal bridges allow heat to enter or escape through a building’s envelope—contributing to energy waste—and can lead to unwanted condensation, moisture damage, expansion/contraction, and freeze-thaw damage.
Structures built to the passive house standard seek to eliminate these thermal bridges wherever possible—such as by installing continuous insulation, use of insulated concrete forms (ICFs) or structural insulated panels (SIPs), and choosing double- or triple-paned windows with thermally-broken frames—to ensure that thermal energy is not wasted one way or another, making it that much easier to passively regulate interior temperatures throughout the year. If thermal bridges cannot be wholly eliminated they must be minimized as much as possible.
Air Control

Passive House-certified buildings typically make use of either a heat recovery ventilation (HRV) or energy recovery ventilation (ERV) system to reduce ventilation-related energy consumption and improve indoor air quality. Photo courtesy of Fantech
Just as they aim to control heat transfer, buildings designed to the passive house standard also seek to effectively control the flow of air that enters and exits the structure. Air control is achieved by air-sealing the building’s envelope and by installing a balanced mechanical ventilation system.
Air-Sealed Building Envelope
Air leakage through gaps and cracks in a building’s envelope accounts for anywhere from 25 to 40% of the energy used for heating and cooling, according to the experts at Energy Star. It is for this reason that structures designed to Passive House standards must employ rigorous air-sealing methods wherever leaks are likely to occur. Air-sealing is so important, in fact, that for a building to even achieve passive house certification, it must first pass a blower door test to ensure it meets the standard’s rigorous airtightness criteria of 0.6 ACH at 1 pound per square foot of pressure (ACH@50 Pa).
Some of the most effective air-sealing measures include the use of high-quality weather-stripping, caulking, liquid flashing membrane, joint and seam fillers, air and water resistant barriers, and stainless steel fabric flashing. PROSOCO is one of the leading manufacturers of air-sealing products and has worked on several passive house projects. The company’s facility houses a custom-engineered testing chamber that is available for external use by engineers, building scientists, and window manufacturers to optimize the air- and water-sealing capacity of their building components.
Balanced Ventilation System
All buildings require some form of ventilation as a means of circulating and refreshing indoor air, and buildings built to passive house standards are no exception. All passive house structures use some form of balanced, whole-building ventilation system that includes heat recovery capabilities, as these systems ensure that the warmth carried by exhaust air is not wasted but instead transferred to incoming fresh air during the winter months, or vice versa during the summer months.
Heat recovery ventilation (HRV) and energy recovery ventilation (ERV) systems are incredibly efficient and are typically capable of recovering at least 75% of the heat from incoming or outgoing air. A ventilation system with balanced heat recovery also helps ensure that a building always receives plentiful, nearly room temperature fresh air year-round, making for a much more comfortable indoor environment and drastically improving indoor air quality.
Solar Radiation Control

Designed by NODE Architecture, Engineering and Consulting, Middle Village is the first Passive House-certified project in Queens. Photo by Brian Berkowitz
Effective solar radiation control, or passive solar design, is a key element of passive house building and informs everything from the project’s orientation and layout to the materials used in the building’s construction. “A hallmark to Passive House design is building with solar orientation, seasonal insulation, and sun path in mind,” Jakov Saric, cofounder and principal architect at NODE Architecture, Engineering and Consulting, wrote in a previous gb&d article. “It’s most favorable to have the southernmost facing part of the building include large windows to allow the low winter sun in the house to provide heat.”
Two of the most common strategies used to control solar radiation are daylighting/solar shading and the installation of high-performance windows.
Daylighting & Solar Shading
Daylighting describes the process of using windows, skylights, and other light-admitting features to effectively illuminate a building’s interior using natural sunlight rather than artificial lighting during daytime hours. When implemented to scale throughout a building, daylighting can help reduce electric lighting loads by as much as 80% and overall building energy consumption by approximately 40%.
Solar shading, on the other hand, refers to those features used to optimize the amount of solar heat that enters a building. Effective solar shading devices serve to block solar heat gain during the hottest months of the year (to prevent overheating) while still allowing sunlight to enter during the coldest months in order to exploit its thermal properties.
Solar shading strategies can be either fixed (stationary) or dynamic (movable). Common fixed solar shading solutions include:
- Light shelves
- Canopies and awnings
- Balconies and overhanging eaves
- External louvers and brise soleil
- Trees (particularly deciduous varieties)
Dynamic shading may be provided by:
- Internal blinds and curtains
- Internal or external shutters
- External roller blinds
- Adjustable shading devices
When implemented correctly, dynamic shading strategies offer a greater amount of control over solar heat gain admittance. Many dynamic shading solutions can even be motorized or automated to respond to external weather conditions.
High-Performance Windows
On average heat lost and gained through windows accounts for approximately 25 to 30% of a building’s total HVAC energy usage, making it extremely important for projects pursuing passive house certification to feature high-performance, energy-efficient windows in their design.
To reduce heat transfer through the glass itself, energy-efficient windows are either double- or triple-paned, with the space between each pane filled with an inert gas—like argon or krypton—that provides added insulation. Likewise, window frames should be constructed from materials with a very low rate of conductivity (e.g. fiberglass) or possess a thermal break to prevent unwanted heat transfer.
Windows used in passive house projects should also feature a low-emissivity film. Low-emissivity coatings are transparent, microscopically thin layers of film that typically contain metallic particles, which help to reflect long-wave infrared energy without compromising visibility. Low-emissivity coatings are the most effective means to lower a window’s solar heat gain coefficient, or the amount of solar radiation that passes through it.
5 Passive House Examples
Here a few examples of passive houses.
1. Acton Passive House, Acton, MA
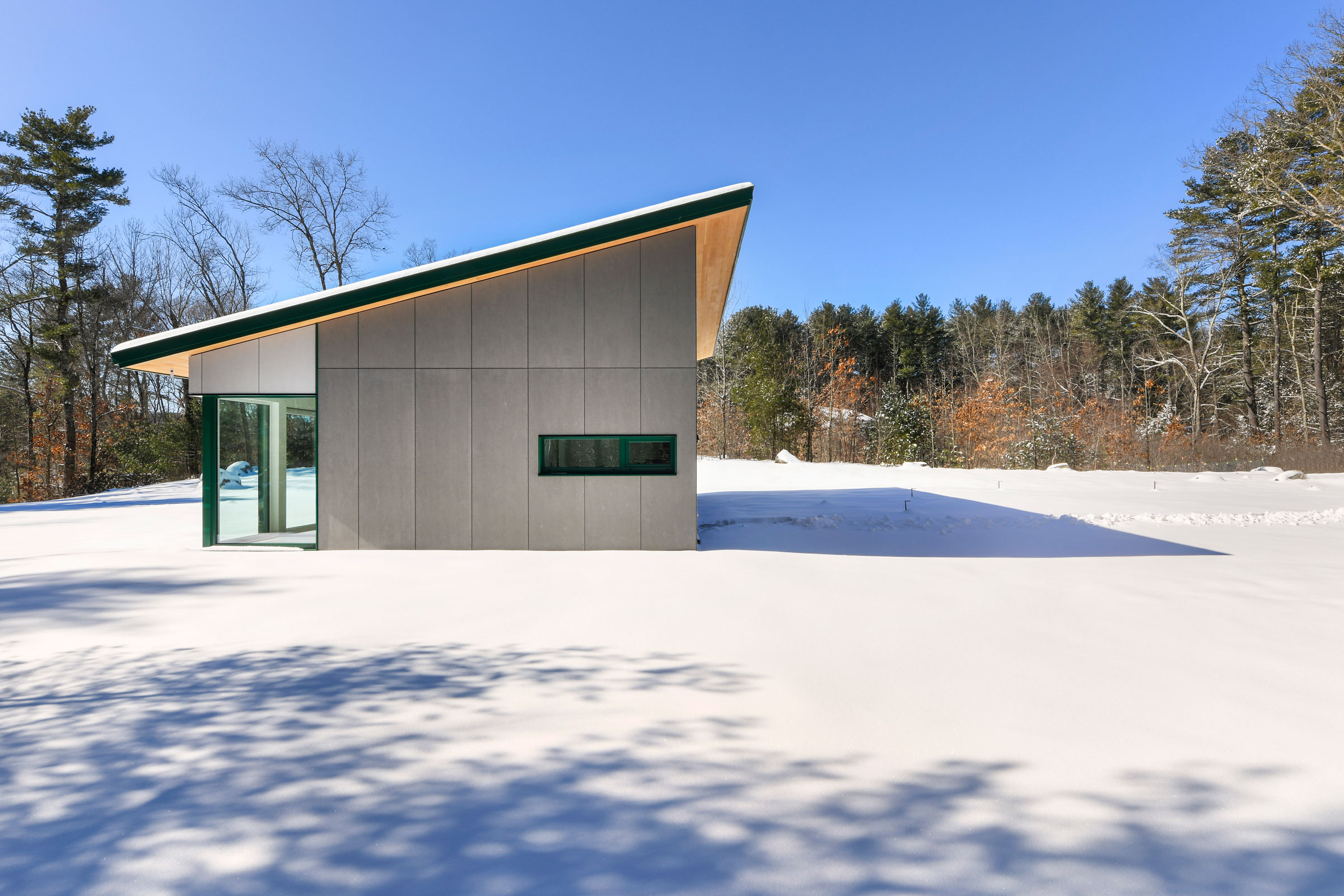
The Acton Passive House designed by ZeroEnergy Design is tucked into a clearing in the woods. Photo by Nat Rea
Nestled into a remote clearing in the woods of Massachusetts, the unassuming Acton Passive House—certified to both the PHIUS and Source-Zero standards—is a simple yet efficient residence that produces all of its own energy via a rooftop solar array.
Designed by Boston-based architecture firm ZeroEnergy Design, the Acton Passive House utilizes dense-packed cellulose insulation wrapped in wood fiberboard—both of which possess a very low or negative embodied carbon—to help passively retain thermal heat energy during the cold winter months. Rigorous air sealing and high-performance windows further serve to reduce the home’s energy consumption.
To minimize the home’s overall environmental impact ZeroEnergy Design elected to construct the Acton Passive House primarily from wood and fiber cement, reducing the amount of concrete required for the build. Both products can be composted or recycled once they reach the end of their operational lifespan, minimizing the amount of landfill waste.
“What we were able to do on this project was rely on a wood structure and minimize the concrete, which typically has the highest carbon impact. Through the process we wanted to make sure we weren’t just looking at operational carbon but also considering the embodied carbon—the carbon that goes into the construction,” Stephanie Horowitz, lead architect and managing director at ZED, told gb&d in a previous article.
2. Charlotte of the Upper West Side, New York City
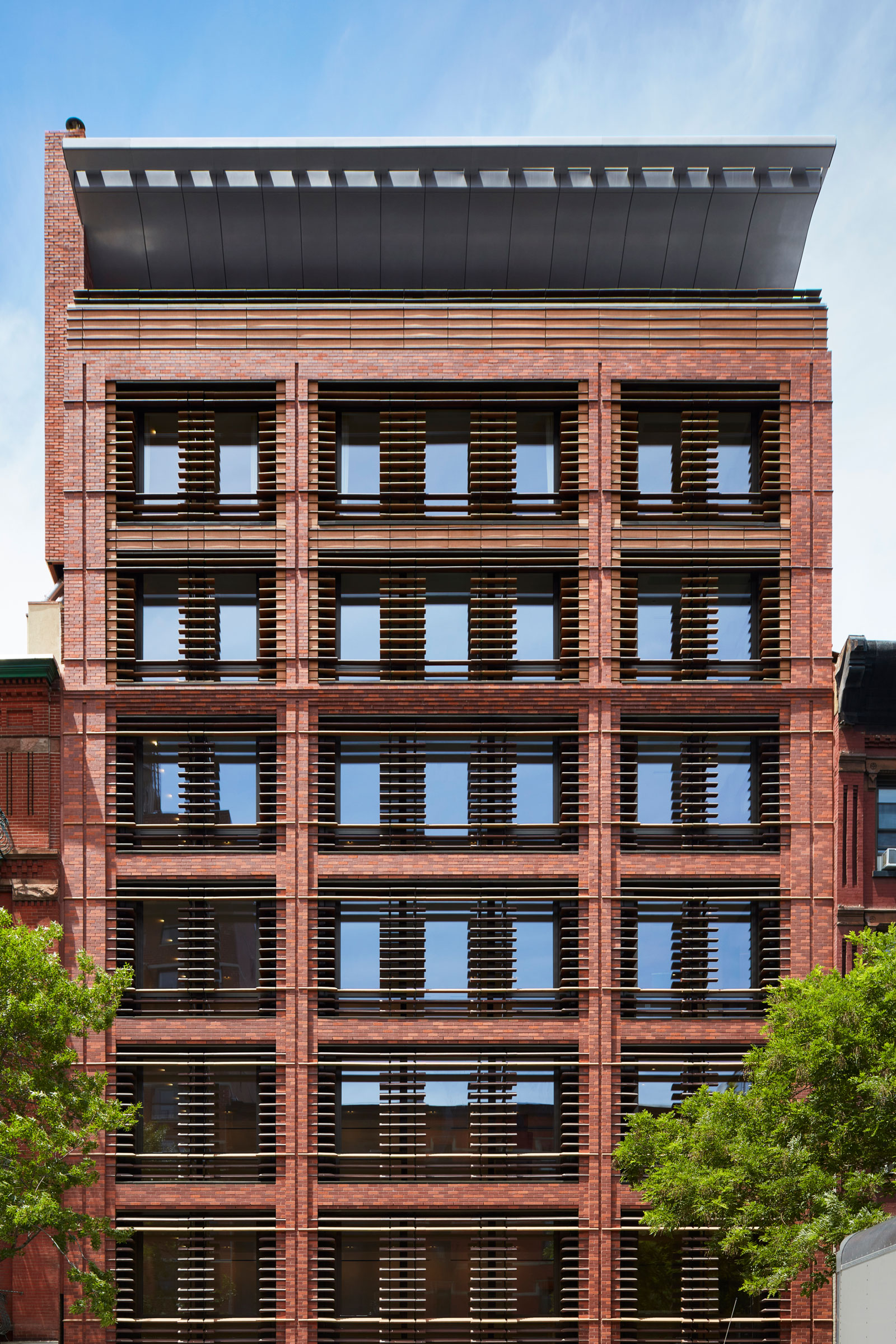
The Charlotte of the Upper West Side condominium complex is among the first of its kind to be constructed in New York City. Photo by Christopher Payne/ESTO
On New York City’s 470 Columbus Avenue, Charlotte of the Upper West Side is one of the first condominium complexes in New York to be constructed to passive house standards.
Designed by BKSK Architects for the Roe Corporation, the mixed-use Charlotte of the Upper West Side housing and retail development is immediately recognizable by its street-facing windows—each featuring triple-paned glass and thermally-broken frames—and custom terra-cotta solar shade and rainscreens. The condominium’s impressive brick walls are highly insulated to ensure thermal comfort year-round.
Each unit in the complex receives filtered fresh air separate from the HVAC system, supplied by a Swiss-engineered Zehnder ERV that facilitates a complete exchange of all interior air inside each apartment 13 times a day. Each ERV is equipped with a MERV 13 filter that offers 30 times the filtering efficiency of standard fiberglass filters and removes everything from pollen and dust to bacteria and tobacco smoke, providing the building with extremely high indoor air quality. “There are many documented health benefits of higher indoor air quality, including a reduced risk of respiratory issues, better sleep, higher productivity, and an overall feeling of well-being,” Todd Poisson, partner at BKSK, previously wrote for gb&d.
Charlotte of the Upper West Side takes its ventilation to the next level, however, by being one of the first residential condominium buildings in New York to use Ultraviolet C (UVC) energy throughout its mechanical ventilation system to irradiate germs and viruses, greatly reducing the spread of air-borne health risks.
In order to comply with PHI’s rigorous air-sealing standards, the team at BKSK sealed off various parts of the building during the construction process for blower door testing, identifying and remedying leaks and weak points as they were discovered.
3. Second + Delaware Apartments, Kansas City, MO
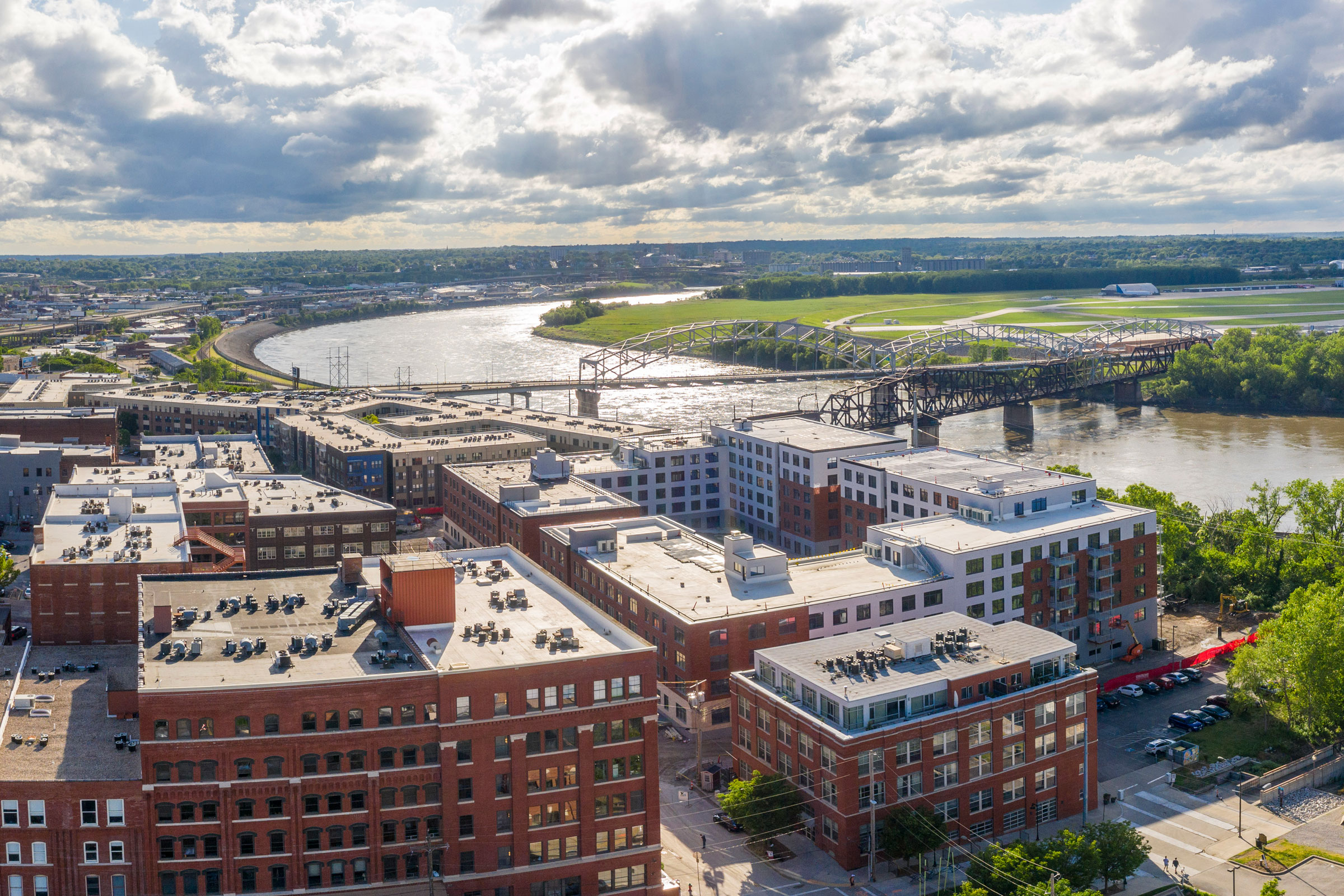
The Second + Delaware Apartments project in Kansas City, Missouri is being called the world’s largest multifamily Passive House development. Photo courtesy of Arnold Development Group
As the world’s largest multifamily Passive House-certified development, the Second + Delaware Apartments in Kansas City, Missouri are an impressive example of how effectively passive systems may be implemented in large-scale projects.
Designed by Jeffrey M. White and developed by the Arnold Development Group, the Second + Delaware Apartments development project encompasses 330,000-square feet and houses 276 apartment units in total. In order to comply with passive house solar radiation control and air-sealing standards, the design team turned to Klearwall—an Irish window manufacturer—and PROSOCO for assistance.
Klearwall supplied the project with highly efficient triple-glazed windows while PROSOCO helped the team figure out the best methods for sealing the window systems to prevent air and water leakage. PROSOCO’s testing chamber allowed the team to try out various solutions, leading to the ultimate decision of installing a fabricated metal sill on top of the window buck and applying PROSOCO’s AirDam product around all four sides.
Additional PROSOCO products—including FastFlash and the company’s Joint & Seam Filler—were used to help effectively seal the rest of the building envelope. All in all, the Second + Delaware Apartments were able to exceed passive house airtightness requirements and are expected to achieve 92% energy savings compared to similar structures.
The apartment complex also features a dedicated heat recovery ventilation system that helps free up roof space for other, more desirable features. “Our roofs are not littered with AC equipment, but are instead amenities like our 14,000-square foot green roof where tenants can garden, and then another 8,000 square feet of a roof terrace with furniture, and a 32,000-square-foot landscaped courtyard, which is approximately the size of a pro soccer field,” Jonathan Arnold, CEO of Arnold Development Group, told gb&d in a previous article.
4. Pine Hall at Wheaton College, Norton, MA
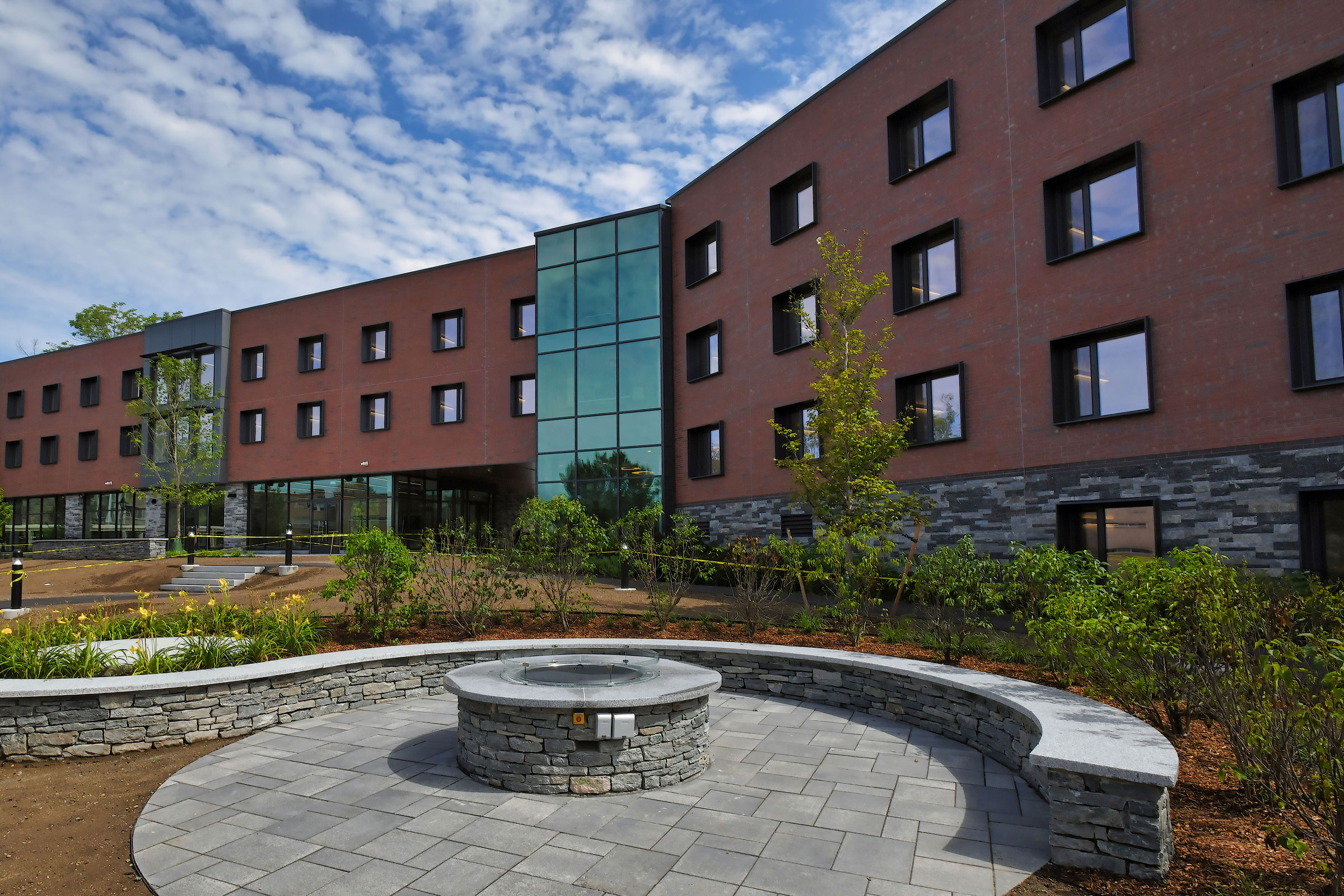
Pine Hall at Wheaton College is the largest Passive House project in New England. Photo courtesy of Wheaton College
Designed by international engineering firm Thornton Tomasetti in collaboration with SGA, Pine Hall at Wheaton College is the first student residence hall in Massachusetts to achieve Passive House-certification and is the largest passive house project in New England.
In order to comply with passive house standards, Pine Hall was designed using airtight, highly insulated construction methods and features triple-glazed energy-efficient windows, R-50 rated roofing materials, and R-32 rated high-performing walls. Exterior shading and high-efficiency heating, cooling, and lighting systems further serve to reduce the building’s energy loads.
“This remarkably efficient building was designed to use up to 50% less energy than a building built to current code and meets Passive House Institute’s (PHIUS) extremely rigorous criteria,” Michael Pulaski, vice president and Portland office director of Thornton Tomasetti, previously told gb&d.
The building itself was constructed with a girder slab framing system and a low-waste steel-and-precast-concrete system that was prefabricated off-site and then assembled onsite. Encompassing 45,000 square feet, the completed Pine Hall is capable of housing 178 students and features a 2,000-square-foot common space designed for programs and events.
5. Bella Bella Passive House, Cambridge Island, Canada
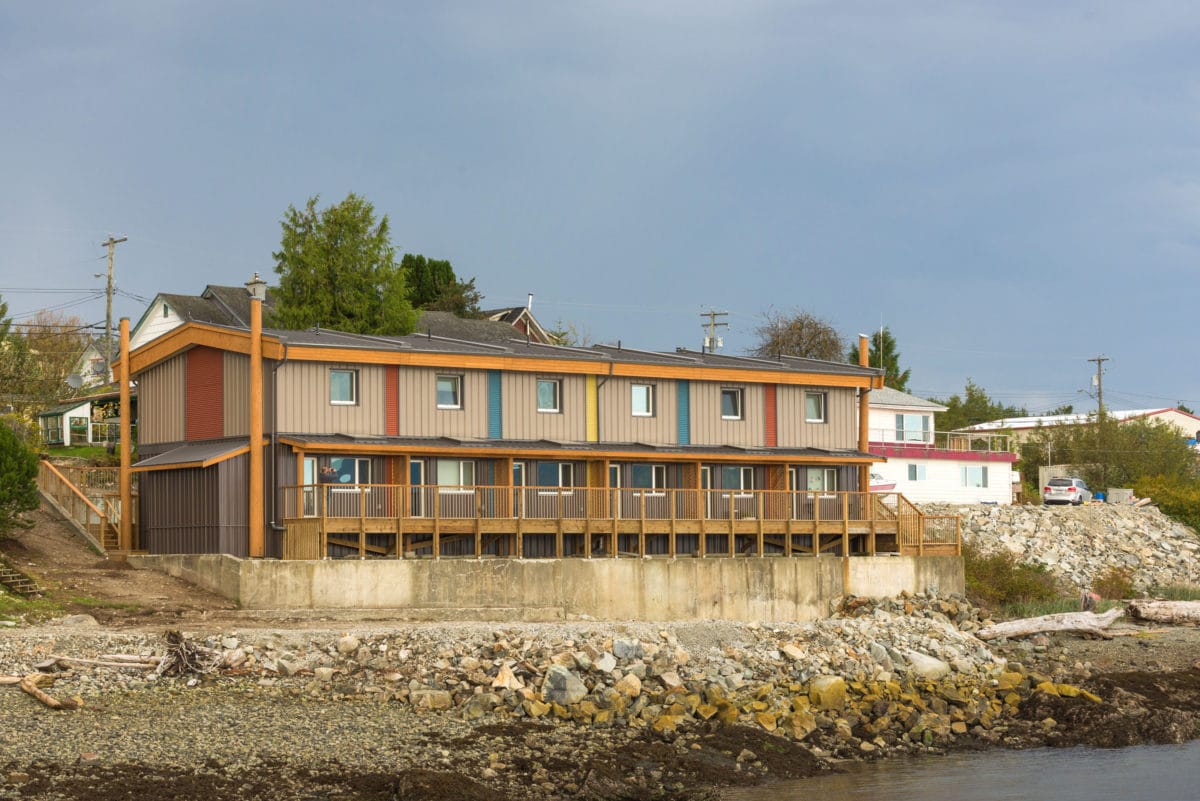
The Bella Bella house for staff of a remote hospital was built to Passive House standards, quickly and efficiently, using prefab techniques. Photo courtesy of Vancouver Coastal Health
Designed for Vancouver Coastal Health’s (VCH) R.W. Large Memorial Hospital by Mobius Architecture, the Bella Bella Passive House showcases that even prefab homes can be built to passive house standards.
After a fire destroyed R.W. Large Memorial Hospital’s staff housing, it was imperative that VCH build a replacement as soon as possible—but because of the hospital’s location on British Columbia’s Campbell Island, a place only accessible by boat or plane, doing so proved easier said than done. Getting materials and a construction team to the remote site would be incredibly challenging, with most new construction projects on the island taking over a year to complete.
To cut construction times, VCH commissioned six two-story attached prefab townhouses from Mobius Architecture to be shipped in from the mainland after prefabrication. “A prefab structure taken up by barge presented a very quick and cost effective way to efficiently meet what would be needed in Bella Bella,” Glen Garrick, sustainability manager, transformation and innovation for VCH, told gb&d in a previous article. “In the end the project was completed at a cost of $2.6 million—about $500,000 less than it would have cost to construct the development onsite.”
Each of the project’s approximately 32 x 14 foot modules—12 in total—were carefully designed by Mobius Architecture and constructed in a Britco facility in the province where they were built and tested to passive house air-tightness standards in a closed, quality-controlled environment. Features such as fiberglass insulation, airtight windows, heat and energy recovery ventilation units, and split-system heat pumps helped each module achieve maximum energy-efficiency while a continuous layer of ROCKWOOL Comfortboard 80 insulation was installed around each module’s exterior to reduce thermal bridging.